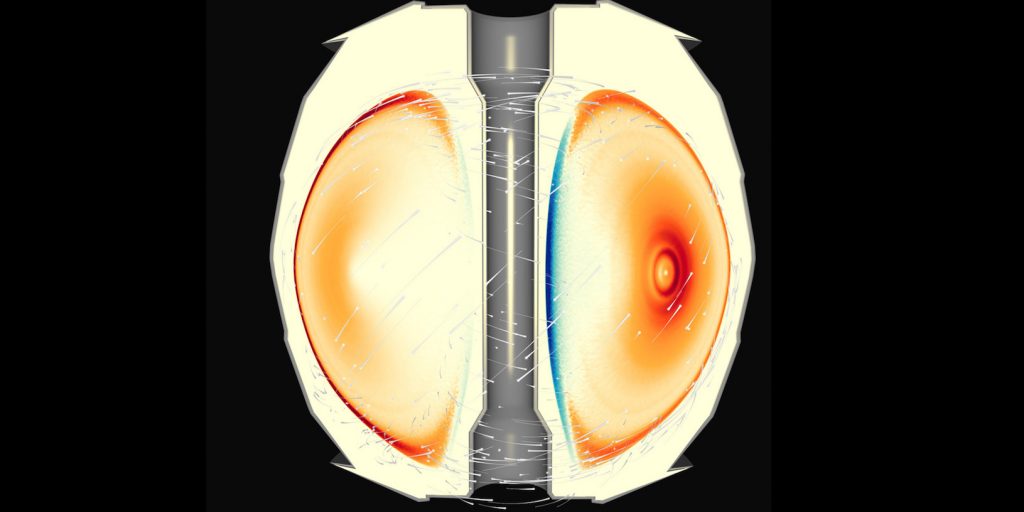
By John Greenwald, PPPL Office of Communications
Researchers at the U.S. Department of Energy’s (DOE) Princeton Plasma Physics Laboratory (PPPL) have challenged understanding of a key element in fusion plasmas. At issue has been an accurate prediction of the size of the “bootstrap current” — a self-generating electric current — and an understanding of what carries the current at the edge of plasmas in doughnut-shaped facilities called tokamaks. This bootstrap-generated current combines with the current in the core of the plasma to produce a magnetic field to hold the hot gas together during experiments, and can produce stability at the edge of the plasma.
The recent work, published in the April issue of the journal Physics of Plasmas, focuses on the region at the edge in which the temperature and density drop off sharply. In this steep gradient region — or pedestal — the bootstrap current is large, enhancing the confining magnetic field but also triggering instability in some conditions.
The bootstrap current appears in a plasma when the pressure is raised. It was first discovered at the University of Wisconsin by Stewart Prager, now director of PPPL, and Michael Zarnstorff, now deputy director for research at PPPL. Prager was Zarnstorff’s thesis advisor at the time.
Physics understanding and accurate prediction of the size of the current at the edge of the plasma is essential for predicting its effect on instabilities that can diminish the performance of fusion reactors. Such understanding will be vital for ITER, the international tokamak under construction in France to demonstrate the feasibility of fusion power. This work was supported by the DOE Office of Science (FES).
The new paper, by physicists Robert Hager and C.S. Chang, leader of the Scientific Discovery through Advanced Computing project’s Center for Edge Physics Simulation headquartered at PPPL, discovered that the bootstrap current in the tokamak edge is mostly carried by the “magnetically trapped” electrons that cannot travel as freely as the “passing” electrons in plasma. The trapped particles bounce between two points in the tokamak while the passing particles swirl all the way around it.
The discovery challenges conventional understanding and provides an explanation of how the bootstrap current can be so large at the tokamak edge, where the passing electron population is small. Previously, physicists thought that only the passing electrons carry the bootstrap current. “Correct modeling of the current enables accurate prediction of the instabilities,” said Hager, the lead author of the paper.
The researchers performed the study by running an advanced global code called “XGCa” on the Mira supercomputer at the Argonne Leadership Computing Facility, a DOE Office of Science User Facility located at the Department’s Argonne National Laboratory. Researchers turned to the new global code, which models the entire plasma volume, because simpler local computer codes can become inadequate and inaccurate in the pedestal region.
Numerous XGCa simulations led Hager and Chang to construct a new formula that greatly improves the accuracy of bootstrap current predictions. The new formula was found to fit well with all the XGCa cases studied and could easily be implemented into modeling or analysis codes.
PPPL, on Princeton University’s Forrestal Campus in Plainsboro, N.J., is devoted to creating new knowledge about the physics of plasmas — ultra-hot, charged gases — and to developing practical solutions for the creation of fusion energy. Results of PPPL research have ranged from a portable nuclear materials detector for anti-terrorist use to universally employed computer codes for analyzing and predicting the outcome of fusion experiments. The Laboratory is managed by the University for the U.S. Department of Energy’s Office of Science, which is the largest single supporter of basic research in the physical sciences in the United States, and is working to address some of the most pressing challenges of our time. For more information, please visit science.energy.gov.
Read the abstract and article.
The paper, “Gyrokinetic neoclassical study of the bootstrap current in the tokamak edge pedestal with fully non-linear Coulomb collisions,” by Robert Hager and C.S. Chang, was published in the April, 2016, Physics of Plasmas, doi: 10.1063/1.4945615.
Support for this work was provided through the Scientific Discovery through Advanced Computing (SciDAC) program funded by the U.S. Department of Energy Office of Advanced Scientific Computing Research and the Office of Fusion Energy Sciences.
You must be logged in to post a comment.