
By the Office of the Dean for Research
The universe has come into sharper focus with the release this week of new images from one of the largest telescopes in the world. A multinational collaboration led by the National Astronomical Observatory of Japan that includes Princeton University scientists has published a “cosmic census” of a large swath of the night sky containing roughly 100 million stars and galaxies, including some of the most distant objects in the universe. These high-quality images allow an unprecedented view into the nature and evolution of galaxies and dark matter.
The images and accompanying data were collected using a digital optical-imaging camera on the Subaru Telescope, located at the Mauna Kea Observatory in Hawaii. The camera, known as Hyper Suprime-Cam, is mounted directly in the optical path, at the “prime focus,” of the Subaru Telescope. A single image from the camera captures an amount of sky equal to the area of about nine full moons.
The project, known as the Hyper Suprime-Cam Subaru Strategic Program, is led by the National Astronomical Observatory of Japan (NAOJ) in collaboration with the Kavli Institute for the Physics and Mathematics of the Universe in Japan, the Academia Sinica Institute of Astronomy and Astrophysics in Taiwan, and Princeton University.
The release includes data from the first one-and-a-half years of the project, consisting of 61.5 nights of observations beginning in 2014. The project will take 300 nights over five to six years.
The data will allow researchers to look for previously undiscovered galaxies and to search for dark matter, which is matter that neither emits nor absorbs light but which can be detected via its effects on gravity. A 2015 study using Hyper Suprime-Cam surveyed 2.3 square degrees of sky and found gravitational signatures of nine clumps of dark matter, each weighing as much as a galaxy cluster (Miyazaki et al., 2015). The current data release covers about 50 times more sky than was used in that study, showing the potential of these data to reveal the statistical properties of dark matter.
The survey consists of three layers: a Wide survey that will eventually cover an area equal to 7000 full moons, or 1400 square degrees; a Deep survey that will look farther into the universe and encompass 26 square degrees; and an UltraDeep survey that will cover 3.5 square degrees and penetrate deep into space, allowing observations of some of the most distant galaxies in the universe. The surveys use optical and near infrared wavelengths in five broad wavelength bands (green, red, infrared, z, and y) and four narrow-band filters. In the multi-band images, the images are extremely sharp, with star images only 0.6 to 0.8 arcseconds across. (One arcsecond equals 3600th part of a degree.)
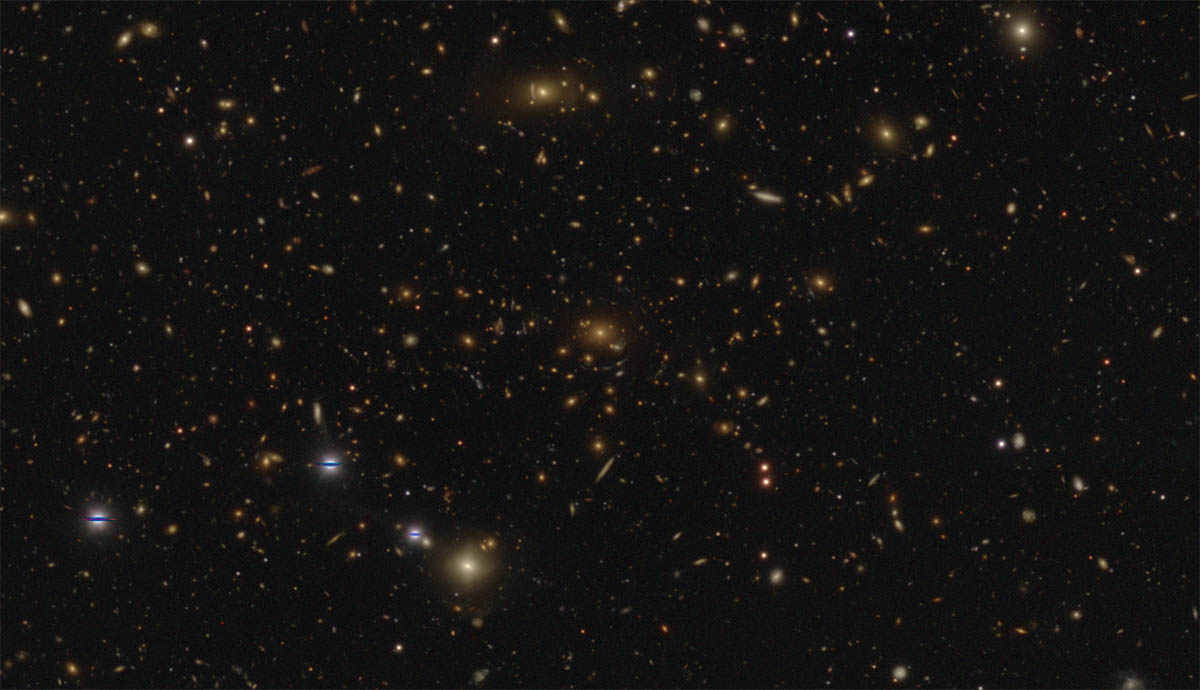
The ability to capture images from deep in space is made possible by the light-collection power of the Subaru Telescope’s mirror, which has an aperture of 8.2 meters, as well as the image exposure time. The depth into space that one can look is measured in terms of the magnitude, or brightness of objects that can be seen from Earth in a given wavelength band. The depths of the three surveys are characterized by magnitudes in the red band of 26.4, 26.6 and 27.3 in the Wide, Deep and Ultradeep data, respectively. As the survey continues, the Deep and Ultradeep surveys will be able to image fainter objects.
The Hyper Suprime-Cam contains 104 scientific charge-coupled devices (CCDs) for a total of 870 million pixels. The total amount of data taken so far comprises 80 terabytes, which is comparable to the size of about 10 million images by a typical digital camera, and covers 108 square degrees. Because it is difficult to search such a huge dataset with standard tools, NAOJ has developed a dedicated database and interface for ease of access and use of the data.
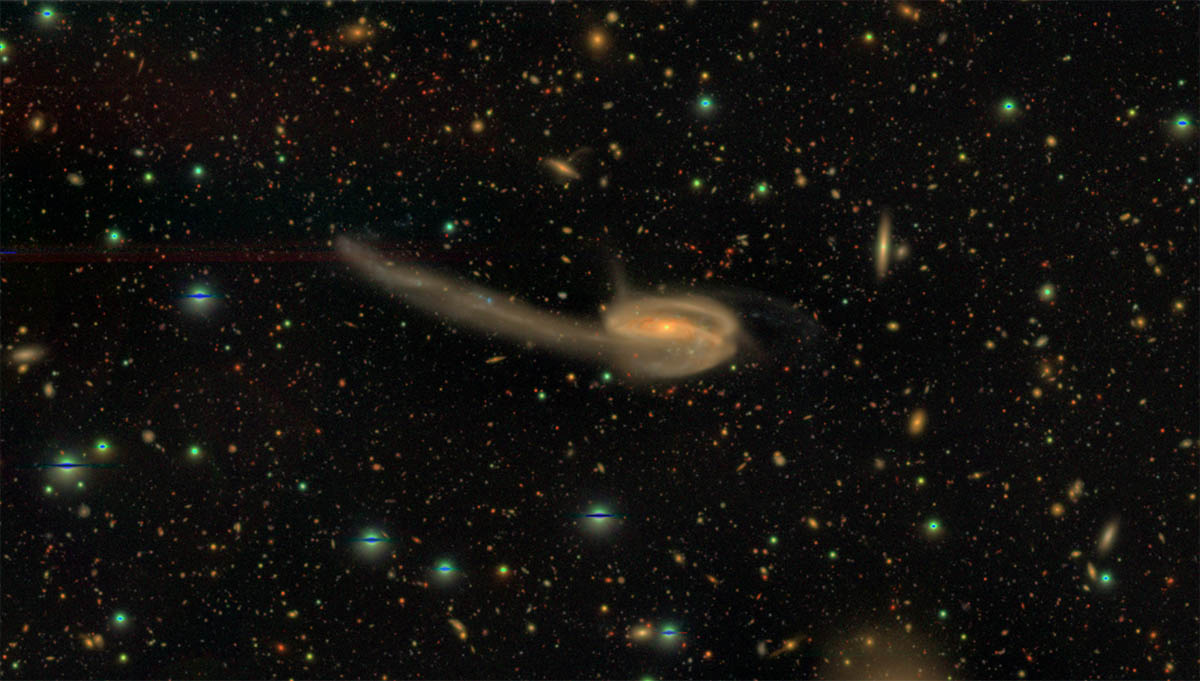
“Since 2014, we have been observing the sky with HSC, which can capture a wide-field image with high resolution,” said Satoshi Miyazaki, the leader of the project and a scientist at NAOJ. “We believe the data release will lead to many exciting astronomical results, from exploring the nature of dark matter and dark energy, as well as asteroids in our own solar system and galaxies in the early universe. The team members are now preparing a number of scientific papers based on these data. We plan to publish them in a special issue of the Publication of Astronomical Society of Japan. Moreover, we hope that interested members of the public will also access the data and enjoy the real universe imaged by the Subaru telescope, one of the largest the world.”
At Princeton, the project is co-led by Michael Strauss and Robert Lupton of the Department of Astrophysical Sciences. “The HSC data are really beautiful,” Strauss said. “Princeton scientists are using these data to explore the nature of merging galaxies, to search for the most distant quasars in the universe, to map the outer reaches of the Milky Way Galaxy, and for many other projects. We are delighted to make these wonderful images available to the world-wide astronomical community.”
Funding for the HSC Project was provided in part by the following grants: Grant-in-Aid for Scientific Research (B) JP15340065; Grant-in-Aid for Scientific Research on Priority Areas JP18072003; and the Funding Program for World-Leading Innovative R&D on Science and Technology (FIRST) entitled, “Uncovering the origin and future of the Universe-ultra-wide-field imaging and spectroscopy reveal the nature of dark matter and dark energy.” Funding was also provided by Princeton University.
This article was adapted from a press release from the National Astronomical Observatory of Japan.
You must be logged in to post a comment.