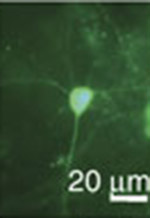
By Morgan Kelly, Office of Communications
Princeton University researchers have created “souped up” versions of the calcium-sensitive proteins that for the past decade or so have given scientists an unparalleled view and understanding of brain-cell communication.
Reported July 18 in the journal Nature Communications, the enhanced proteins developed at Princeton respond more quickly to changes in neuron activity, and can be customized to react to different, faster rates of neuron activity. Together, these characteristics would give scientists a more precise and comprehensive view of neuron activity.
The researchers sought to improve the function of proteins known as green fluorescent protein/calmodulin protein (GCaMP) sensors, an amalgam of various natural proteins that are a popular form of sensor proteins known as genetically encoded calcium indicators, or GECIs. Once introduced into the brain via the bloodstream, GCaMPs react to the various calcium ions involved in cell activity by glowing fluorescent green. Scientists use this fluorescence to trace the path of neural signals throughout the brain as they happen.
GCaMPs and other GECIs have been invaluable to neuroscience, said corresponding author Samuel Wang, a Princeton associate professor of molecular biology and the Princeton Neuroscience Institute. Scientists have used the sensors to observe brain signals in real time, and to delve into previously obscure neural networks such as those in the cerebellum. GECIs are necessary for the BRAIN Initiative President Barack Obama announced in April, Wang said. The estimated $3 billion project to map the activity of every neuron in the human brain cannot be done with traditional methods, such as probes that attach to the surface of the brain. “There is no possible way to complete that project with electrodes, so you have to do it with other tools — GECIs are those tools,” he said.
Despite their value, however, the proteins are still limited when it comes to keeping up with the fast-paced, high-voltage ways of brain cells, and various research groups have attempted to address these limitations over the years, Wang said.
“GCaMPs have made significant contributions to neuroscience so far, but there have been some limits and researchers are running up against those limits,” Wang said.
One shortcoming is that GCaMPs are about one-tenth of a second slower than neurons, which can fire hundreds of times per second, Wang said. The proteins activate after neural signals begin, and mark the end of a signal when brain cells have (by neuronal terms) long since moved on to something else, Wang said. A second current limitation is that GCaMPs can only bind to four calcium ions at a time. Higher rates of cell activity cannot be fully explored because GCaMPs fill up quickly on the accompanying rush of calcium.
The Princeton GCaMPs respond more quickly to changes in calcium so that changes in neural activity are seen more immediately, Wang said. By making the sensors a bit more sensitive and fragile — the proteins bond more quickly with calcium and come apart more readily to stop glowing when calcium is removed — the researchers whittled down the roughly 20 millisecond response time of existing GCaMPs to about 10 milliseconds, Wang said.
The researchers also tweaked certain GCaMPs to be sensitive to different types of calcium ion concentrations, meaning that high rates of neural activity can be better explored. “Each probe is sensitive to one range or another, but when we put them together they make a nice choir,” Wang said.
The researchers’ work also revealed the location of a “bottleneck” in GCaMPs that occurs when calcium concentration is high, which poses a third limitation of the existing sensors, Wang said. “Now that we know where that bottle neck is, we think we can design the next generation of proteins to get around it,” Wang said. “We think if we open up that bottleneck, we can get a probe that responds to neuronal signals in one millisecond.”
The faster protein that the Princeton researchers developed could pair with work in other laboratories to improve other areas of GCaMP function, Wang said. For instance, a research group out of the Howard Hughes Medical Institute reported in Nature July 17 that it developed a GCaMP with a brighter fluorescence. Such improvements on existing sensors gradually open up more of the brain to exploration and understanding, said Wang, adding that the Princeton researchers will soon introduce their sensor into fly and mammalian brains.
“At some level, what we’ve done is like taking apart an engine, lubing up the parts and putting it back together. We took what was the best version of the protein at the time and made changes to the letter code of the protein,” Wang said. “We want to watch the whole symphony of thousands of neurons do their thing, and we think this variant of GCaMPs will help us do that better than anyone else has.”
Sun, Xiaonan R., Aleksandra Badura, Diego A. Pacheco, Laura A. Lynch, Eve R. Schneider, Matthew P. Taylor, Ian B. Hogue, Lynn W. Enquist, Mala Murthy and Samuel S.-H. Wang. 2013. Fast GCaMPs for improved tracking of neuronal activity. Nature Communications. Article first published online: July 18, 2013. DOI: 10.1038/ncomms3170
This work was supported by NIH R01 NS045193, (S.S.-H.W.) RC1 NS068414 (L.W.E./S.S.-H.W.), and P40 RR18604 and NS060699 (L.W.E.), a McKnight Technological Innovations Award (S.S.-H.W.), a W.M. Keck Foundation Distinguished Young Investigator award (S.S.-H.W.), an Alfred P. Sloan Research Fellowship, Klingenstein, McKnight, and NSF CAREER Young Investigator awards (M.M.), and an American Cancer Society Postdoctoral Research Fellowship (M.P.T./I.B.H.).
You must be logged in to post a comment.