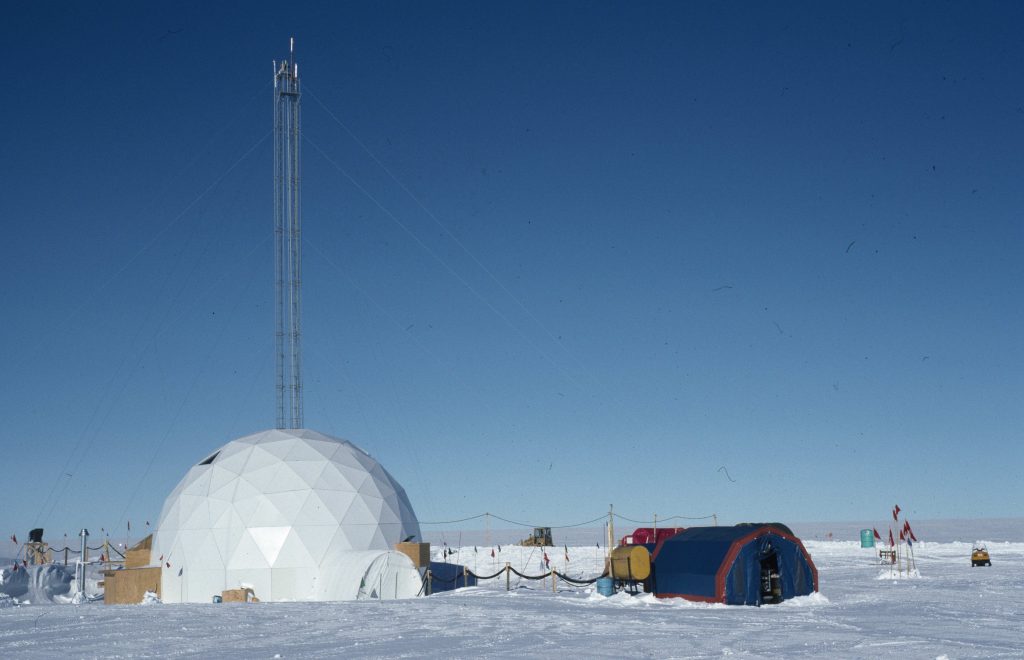
By Morgan Kelly, Office of Communications
Princeton University researchers have compiled 30 years of data to construct the first ice core-based record of atmospheric oxygen concentrations spanning the past 800,000 years, according to a paper published today in the journal Science.
The record shows that atmospheric oxygen has declined 0.7 percent relative to current atmospheric-oxygen concentrations, a reasonable pace by geological standards, the researchers said. During the past 100 years, however, atmospheric oxygen has declined by a comparatively speedy 0.1 percent because of the burning of fossil fuels, which consumes oxygen and produces carbon dioxide.
Curiously, the decline in atmospheric oxygen over the past 800,000 years was not accompanied by any significant increase in the average amount of carbon dioxide in the atmosphere, though carbon dioxide concentrations do vary over individual ice age cycles. To explain this apparent paradox, the researchers called upon a theory for how the global carbon cycle, atmospheric carbon dioxide and Earth’s temperature are linked on geologic timescales.
“The planet has various processes that can keep carbon dioxide levels in check,” said first author Daniel Stolper, a postdoctoral research associate in Princeton’s Department of Geosciences. The researchers discuss a process known as silicate weathering in particular, wherein carbon dioxide reacts with exposed rock to produce, eventually, calcium carbonate minerals, which trap carbon dioxide in a solid form. As temperatures rise due to higher carbon dioxide in the atmosphere, silicate-weathering rates are hypothesized to increase and remove carbon dioxide from the atmosphere faster.

Stolper and his co-authors suggest that the extra carbon dioxide emitted due to declining oxygen concentrations in the atmosphere stimulated silicate weathering, which stabilized carbon dioxide but allowed oxygen to continue to decline.
“The oxygen record is telling us there’s also a change in the amount of carbon dioxide [that was created when oxygen was removed] entering the atmosphere and ocean,” said co-author John Higgins, Princeton assistant professor of geosciences. “However, atmospheric carbon dioxide levels aren’t changing because the Earth has had time to respond via increased silicate-weathering rates.
“The Earth can take care of extra carbon dioxide when it has hundreds of thousands or millions of years to get its act together. In contrast, humankind is releasing carbon dioxide today so quickly that silicate weathering can’t possibly respond fast enough,” Higgins continued. “The Earth has these long processes that humankind has short-circuited.”
The researchers built their history of atmospheric oxygen using measured ratios of oxygen-to-nitrogen found in air trapped in Antarctic ice. This method was established by co-author Michael Bender, professor of geosciences, emeritus, at Princeton.
Because oxygen is critical to many forms of life and geochemical processes, numerous models and indirect proxies for the oxygen content in the atmosphere have been developed over the years, but there was no consensus on whether oxygen concentrations were rising, falling or flat during the past million years (and before fossil fuel burning). The Princeton team analyzed the ice-core data to create a single account of how atmospheric oxygen has changed during the past 800,000 years.
“This record represents an important benchmark for the study of the history of atmospheric oxygen,” Higgins said. “Understanding the history of oxygen in Earth’s atmosphere is intimately connected to understanding the evolution of complex life. It’s one of these big, fundamental ongoing questions in Earth science.”
Daniel A. Stolper, Michael L. Bender, Gabrielle B. Dreyfus, Yuzhen Yan, and John A. Higgins. 2016. A Pleistocene ice core record of atmospheric oxygen concentrations. Science. Article published Sept. 22, 2016. DOI: 10.1126/science.aaf5445
The work was supported by a National Oceanic and Atmospheric Administration Climate and Global Change postdoctoral fellowship, and the National Science Foundation (grant no. ANT-1443263).
You must be logged in to post a comment.