By Catherine Zandonella, Office of the Dean for Research
It’s a beautiful theory: the standard model of cosmology describes the universe using just six parameters. But it is also strange. The model predicts that dark matter and dark energy – two mysterious entities that have never been detected — make up 95% of the universe, leaving only 5% composed of the ordinary matter so essential to our existence.
In an article in this week’s Science, Princeton astrophysicist David Spergel reviews how cosmologists came to be certain that we are surrounded by matter and energy that we cannot see. Observations of galaxies, supernovae, and the universe’s temperature, among other things, have led researchers to conclude that the universe is mostly uniform and flat, but is expanding due to a puzzling phenomenon called dark energy. The rate of expansion is increasing over time, counteracting the attractive force of gravity. This last observation, says Spergel, implies that if you throw a ball upward you will see it start to accelerate away from you.
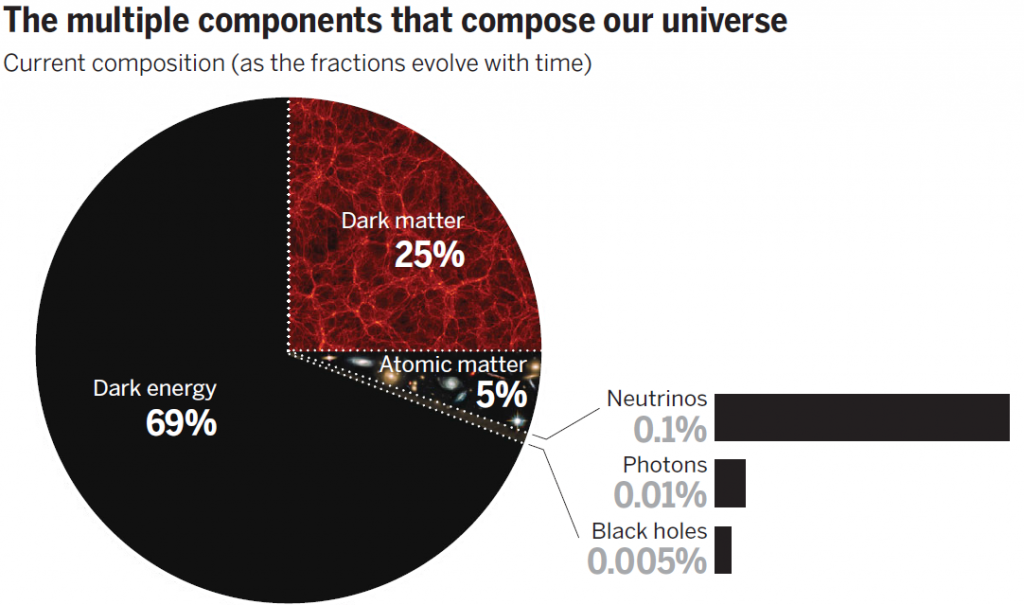
A number of experiments to detect dark matter and dark energy are underway, and some researchers have already claimed to have found particles of dark matter, although the results are controversial. New findings expected in the coming years from the Large Hadron Collider, the world’s most powerful particle accelerator, could provide evidence for a proposed theory, supersymmetry, that could explain the dark particles.
But explaining dark energy, and why the universe is accelerating, is a tougher problem. Over the next decade, powerful telescopes will come online to map the structure of the universe and trace the distribution of matter over the past 10 billion years, providing new insights into the source of cosmic acceleration.
Yet observations alone are probably not enough, according to Spergel. A full understanding will require new ideas in physics, perhaps even a new theory of gravity, possibly including extra dimensions, Spergel writes. “We will likely need a new idea as profound as general relativity to explain these mysteries.”
When that happens, our understanding of the dark side of cosmology will no longer accelerate away from us.
Citation: Spergel, David. The dark side of cosmology: Dark matter and dark energy. Science, 6 March 2015: Vol. 347 no. 6226 pp. 1100-1102 DOI: 10.1126/science.aaa0980.
–David Spergel is the Charles A. Young Professor of Astronomy on the Class of 1897 Foundation, a professor of astrophysical sciences, and chair of Princeton’s Department of Astrophysical Sciences. His research is supported by the National Science Foundation and NASA.
You must be logged in to post a comment.