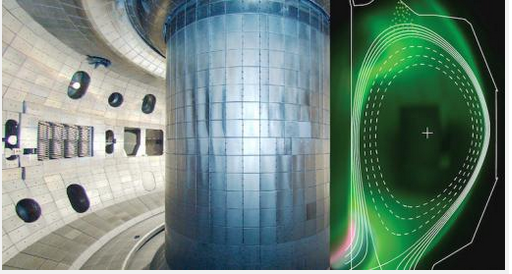
By Raphael Rosen, Princeton Plasma Physics Laboratory
Scientists from General Atomics and the U.S. Department of Energy’s (DOE) Princeton Plasma Physics Laboratory (PPPL) have discovered a phenomenon that helps them to improve fusion plasmas, a finding that may quicken the development of fusion energy. Together with a team of researchers from across the United States, the scientists found that when they injected tiny grains of lithium into a plasma undergoing a particular kind of turbulence then, under the right conditions, the temperature and pressure rose dramatically. High heat and pressure are crucial to fusion, a process in which atomic nuclei – or ions – smash together and release energy — making even a brief rise in pressure of great importance for the development of fusion energy.
“These findings might be a step towards creating our ultimate goal of steady-state fusion, which would last not just for milliseconds, but indefinitely,” said Tom Osborne, a physicist at General Atomics and lead author of the paper. This work was supported by the DOE Office of Science.
The scientists used a device developed at PPPL to inject grains of lithium measuring some 45 millionths of a meter in diameter into a plasma in the DIII-D National Fusion Facility – or tokamak – that General Atomics operates for DOE in San Diego. When the lithium was injected while the plasma was relatively calm, the plasma remained basically unaltered. Yet as reported this month in a paper in Nuclear Fusion, when the plasma was undergoing a kind of turbulence known as a “bursty chirping mode,” the injection of lithium doubled the pressure at the outer edge of the plasma. In addition, the length of time that the plasma remained at high pressure rose by more than a factor of 10.
Experiments have sustained this enhanced state for up to one-third of a second. A key scientific objective will be to extend this enhanced performance for the full duration of a plasma discharge.
Physicists have long known that adding lithium to a fusion plasma increases its performance. The new findings surprised researchers, however, since the small amount of lithium raised the plasma’s temperature and pressure more than had been expected.
These results “could represent the birth of a new tool for influencing or perhaps controlling tokamak edge physics,” said Dennis Mansfield, a physicist at PPPL and a coauthor of the paper who helped develop the injection device called a “lithium dropper.” Also working on the experiments were researchers from Lawrence Livermore National Laboratory, Oak Ridge National Laboratory, the University of Wisconsin-Madison and the University of California-San Diego.
Conditions at the edge of the plasma have a profound effect on the superhot core of the plasma where fusion reactions take place. Increasing pressure at the edge region raises the pressure of the plasma as a whole. And the greater the plasma pressure, the more suitable conditions are for fusion reactions. “Making small changes at the plasma’s edge lets us increase the pressure further within the plasma,” said Rajesh Maingi, manager of edge physics and plasma-facing components at PPPL and a coauthor of the paper.
Further experiments will test whether the lithium’s interaction with the bursty chirping modes — so-called because the turbulence occurs in pulses and involves sudden changes in pitch — caused the unexpectedly strong overall effect.
PPPL, on Princeton University’s Forrestal Campus in Plainsboro, N.J., is devoted to creating new knowledge about the physics of plasmas — ultra-hot, charged gases — and to developing practical solutions for the creation of fusion energy. Results of PPPL research have ranged from a portable nuclear materials detector for anti-terrorist use to universally employed computer codes for analyzing and predicting the outcome of fusion experiments. The Laboratory is managed by the University for the U.S. Department of Energy’s Office of Science, which is the largest single supporter of basic research in the physical sciences in the United States, and is working to address some of the most pressing challenges of our time. For more information, please visit science.energy.gov.
T.H. Osborne, G.L. Jackson, Z. Yan, R. Maingi, D.K. Mansfield, B.A. Grierson, C.B. Chrobak, A.G. McLean, S.L. Allen, D.J. Battaglia, A.R. Briesemeister, M.E. Fenstermacher, G.R. McKee, P.B. Snyder and The DIII-D Team. “Enhanced H-mode pedestals with lithium injection in DIII-D.” Nuclear Fusion. Published May 8, 2015. DOI:10.1088/0029-5515/55/6/063018
You must be logged in to post a comment.