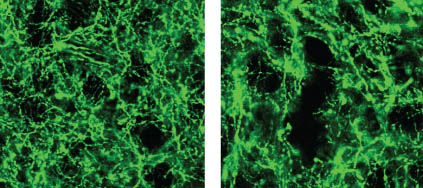
By Catherine Zandonella, Office of the Dean for Research
Princeton University researchers have found that dopamine – a brain chemical involved in learning, motivation and many other functions – also has a direct role in representing or encoding movement. The finding could help researchers better understand dopamine’s role in movement-related disorders such as Parkinson’s disease.
The researchers used a new, more precise technique to record the activity of dopamine neurons at two regions within a part of the brain known as the striatum, which oversees action planning, motivation and reward perception. The researchers found that while all of the neurons carried signals needed to learn and plan movement, one of the nerve bundles, the one that went to the region called the dorsomedial striatum, also carried a signal that could be used to control movements.
The work was published online in the journal Nature Neuroscience this week.
“What we learned from this study is that dopamine neurons that go to one part of the brain act differently than dopamine neurons that go to another part of the brain,” said Ilana Witten, assistant professor of psychology and the Princeton Neuroscience Institute. “This is contrary to what has been the mainstream view of dopamine neurons.”
The research may shed light on how Parkinson’s disease, which involves the destruction of dopamine neurons in the dorsomedial striatum, deprives patients of the ability to move. Previous studies have failed to find a direct link between dopamine neuron activity and the control of movement or actions. Instead, the mainstream view suggested an indirect role for dopamine: the neurons make it possible for us to learn which actions are likely to lead to a rewarding experience, which in turn enables us to plan to take that action. When dopamine neurons are destroyed, the individual cannot learn to plan actions and thus cannot move.
The new study affirmed the role of dopamine in reward-based learning, but also found that in the dorsomedial striatum, dopamine neurons can play a direct role in movement. The researchers used a method for measuring neuron activity at very precise locations in the brain. They measured the activity at the ends of neurons – the terminals where dopamine is released into the junction, or synapse, between two cells – in two locations in the striatum: the nucleus accumbens, known to be involved in processing reward, and the dorsomedial striatum, known for evaluating and generating actions.
Until recently, it has been difficult to measure dopamine neuron activity in these regions due to the small size of the regions and the fact that there are many other neurons present that are delivering other brain chemicals, or neurotransmitters, to the same areas of the brain.
To restrict their measurements to only dopamine-carrying neurons, the researchers used mice whose brains carry genetically altered cells that glow green when active. The mice also contain a second gene that ensured that the glowing could only occur when dopamine was present.
The researchers then recorded neuron activity from either the nucleus accumbens or the dorsomedial striatum by inserting a very thin optical fiber into each region to record the fluorescing dopamine cells in only the desired regions.
Once the ability to measure neuron activity was in place, the researchers gave the mice a task that involved both reward-based learning as well as movement.
The task involved presenting the mice with two levers, one of which, when pressed, gave a drink of sweetened water. Through trial and error, the mice learned which lever would give the reward. During the task, the researchers recorded their brain activity.
The task is analogous to playing slot machines at a casino. Picture yourself at a casino with two slot machines in front of you. You pull the lever on the machine to your left and it spits out some coins. Your brain learns that the left lever leads to a reward, so you plan and execute an action: you pull the left lever again. After a few more pulls on the left lever without a reward, you switch to the machine on the right.
When an action is rewarding, you are likely to remember it, an important step in learning. The difference between how much reward you expect, and how much you get, is also important, because it tells you whether or not something is new and how much you should pay attention to it. Researchers call this gap between your predicted reward and the reward you actually get the “reward-prediction error” and consider it an important teaching signal.
By matching the mice’s actions to the dopamine activity in their brains during these tasks, the researchers could determine which parts of the brain were active during reward-based learning, and which parts were active when choosing to press a lever. Assistance with computational modeling of the mice’s behaviors was provided by Nathaniel Daw, a professor of the Princeton Neuroscience Institute and Psychology.
The researchers found that the dopamine neurons that innervate the nucleus accumbens and the dorsomedial striatum did indeed encode reward-prediction cues, which is consistent with previous findings. But they also found that in the dorsomedial striatum, the dopamine neurons carried information about what actions the animal is going to take.
“This idea was that dopamine neurons carry this reward-prediction error signal, and that could indirectly affect movement or actions, because if you don’t have this, you won’t correctly learn which actions to perform,” Witten said. “We show that while this is true, it is certainly not the whole story. There is also a layer where dopamine is directly coding movement or actions.”
Nathan Parker, a graduate student in the Witten lab who designed and conducted the experiments and is first author on the paper, added that new findings were made possible both by the improvements in recording of neurons and by the experimental design, which gave researchers a detailed evaluation of neuron activity during a relatively complex task.
Additional research assistance was provided by Princeton postdoctoral research associates Courtney Cameron and Junuk Lee, and graduate student Jung Yoon Choi. Research Specialist Joshua Taliaferro, Class of 2015, begin working on the project as part of his senior thesis. The study also involved contributions from Thomas Davidson, a postdoctoral researcher at Stanford University.
The study also addresses the general question of how dopamine can be involved in so many functions in the brain, Witten said. “We think that some of the way that dopaminergic neurons achieve such diverse functions in the brain is by having specific roles based on their anatomical target.”
Naoshige Uchida, a professor of molecular and cellular biology at Harvard University who was not involved in the study, said the results challenge long-held views and open up new directions of research. “This study by the Witten lab elegantly shows that the activity of some dopamine neurons is modulated by the direction of motion,” Uchida said. “More importantly, they found some of the clearest evidence indicating the heterogeneity of dopamine neurons: A specific population of dopamine neurons projecting to the dorsomedial striatum encodes movement direction more so compared to another population projecting to the ventral striatum.”
Uchida continued, “A similar phenomenon has also been reported in an independent study in non-human primates (Kim, et al., Cell, 2015), suggesting that the Witten lab finding is more universal and not specific to mice. This is particularly important because dopamine has been implicated in Parkinson’s disease but how dopamine regulates movement remains a large mystery.”
Funding for the study was provided by the Pew Charitable Trusts, the McKnight Foundation, the Brain & Behavior Research (NARSAD) Foundation, the Alfred P. Sloan Foundation, the National Institutes of Health, the National Science Foundation, and Princeton’s Stuart M. Essig ’83 and Erin S. Enright ’82 Fund for Innovation in Engineering and Neuroscience.
The study, “Reward and choice encoding in terminals of midbrain dopamine neurons depends on striatal target,” by Nathan Parker, Courtney Cameron, Joshua Taliaferro, Junuk Lee, Jung Yoon Choi, Thomas Davidson, Nathaniel Daw and Ilana Witten, was published online in the journal Nature Neuroscience (Advance Online Publication, http://dx.doi.org/10.1038/nn.4287).
You must be logged in to post a comment.